In contrast to gold-, silver- and copper-coloured coins (whatever metal they are made of), simply “coloured coins” sport the colours not usually associated with coinage metals. Or so I think, because so far I could not find any definitive guide to coloured coins. Wikipedia mentions them in an article on
commemorative coins. I got interested in the subject since I discovered that the Sherlock Holmes Silver Coins produced by the
New Zealand Mint (apparently, the legal tender of the
Cook Islands) are graced by the images of Sherlock Holmes and Doctor Watson from Soviet-era films, such as
The Hound of the Baskervilles.
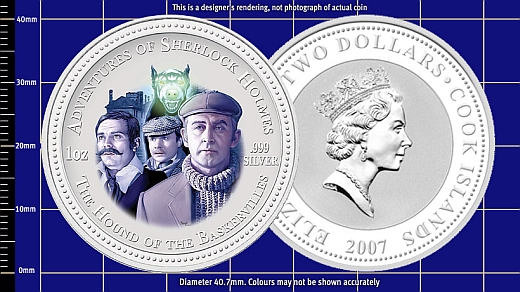
The
Tony Clayton’s website contains a very useful
list of metals used in coins and medals. In particular, I have learned that
niobium is used as coinage metal. In 2003,
Münze Österreich pioneered the use of niobium for coin manufacturing, issuing a
bimetallic €25 coin. According to
this review,
The colouring <of the niobium insert> is made by a so called anodic oxidation of the material. With this treatment, by electrochemical processing a very thin niobium oxide layer is formed under controlled conditions. By refraction of light in the oxide layer so called interference colours are created which gives the colouring of the niobium. Depending on the processing parameters, the thickness of the oxide layer can be very well controlled, and gives the niobium its noble appearance. Depending on the thickness of the layer different colours are producible.
For instance, Latvian bimetallic
Coin of Time (struck by Münze Österreich) consists of beautiful blue niobium centre enclosed in an outer silver ring. The obverse of the coin features the heraldic rose and the tiny Gothic script letters
ℌ and
ℜ standing for
Heinrich Rose (1795—1864), discoverer of niobium.